Listening to the brain with higher precision and less damage
We need to be able to listen to the brain in order to better understand how the brain works and what happens when it is affected by different conditions. We must be able to do this without causing damage, while being certain about which brain cells we are actually listening to. Only then can we study which cells are active during chronic pain or follow what actually happens when someone suffers from conditions such as Alzheimer’s disease or depression. With increased precision we can also stimulate the brain in a more exact manner and with considerably fewer side effects than today, for example to inhibit tremors in Parkinson’s disease. There will soon be brain electrodes that meet these requirements.
“We need to develop electrodes that make it possible to study the brain and, ideally, individual cells without damaging them. The aim is to learn how the brain works and how things fit together. This will open up entirely new possibilities for treatment”, says Jens Schouenborg, neurophysiologist and professor at Lund University’s Neuronano Research Centre.
The problem with most of the electrodes used today is that they are stiff and often quite rough, which means they irritate the brain tissue when the brain moves, which it does more or less the whole time, such as when we breathe or move our head. The electrodes rub against the surrounding brain tissue, which leads to scarring around the electrode known as a “kill-zone” of dead nerve cells – the outcome is that you have damaged what you want to study.
Jens Schouenborg and his research group have been working for a long time on developing thinner and more flexible electrodes that can follow the brain’s natural movements. It was shown that thin, flexible electrodes with approximately the same density as brain tissue caused the least damage. However, the electrodes are so thin that they cannot even break the surface tension of water. The electrodes are embedded in gelatine to enable them to be implanted in the brain. The gelatine is gentle on the brain, does not cause scarring and dissolves once the electrode is in place.
Tests so far have only been carried out on rats, but the new technique opens up possibilities for registering what happens in the brain of an animal that is awake and moving, and for doing so for a long period, up to several months.
The results were recently published (Sept 2015) in the research journal, Frontiers in Neuroscience.
“This was a breakthrough for flexible implants. We succeeded in showing that super-thin flexible electrodes can be implanted in the brain without damaging it, and that kill-zones are practically non-existent around this type of implant. We have also shown that the electrodes work and retain their structure. The latter is a prerequisite for enabling us to register signals from the same cells over a long period.”
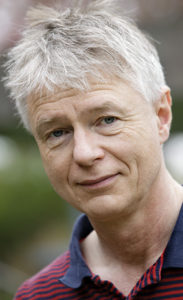
Jens Schouenborg, neurophysiologist and professor at Lund University’s Neuronano Research Centre.
So far the researchers have worked on a microscale, but now when there is a functioning method using flexible electrodes that do not damage the brain, there is increasing interest in trying to make it work on a nanoscale. Nano, which is one millionth of a millimetre, provides opportunities to study various processes in more detail. Regarding nerve cells it opens up possibilities, for example to undertake detailed studies of nerve cells’ synapses (the interface between two nerve cells where signal transfers take place).
“At the moment we are working on the nanostructuring of the super-thin, flexible electrodes”, says Jens Schouenborg, somewhat cryptically.
He is not prepared to reveal any more at the moment. He can, however, say that in cooperation with physicists at the Neuronano Research Centre it has been possible to make and implant a nanoelectrode, an electrode with super-thin nanowires, in the brain of a rat, and that signals were successfully captured from the nerve cells. The remaining challenge is to make the nanowire-based electrodes as flexible as those on a microscale.
Super-thin flexible electrodes that can be placed exactly where the researchers want them and that register signals from single, or a few, cells are interesting tools for basic research, but what medical applications are in the pipeline?
The stimulation of different areas in the brain is of clinical interest. The method has been applied for quite long periods in patients with Parkinson’s disease in order to reduce the tremors associated with the condition. The problem with these electrodes is that they are quite rough (in millimetre scale) and stiff, which leads to scarring in the brain. This in turn means that a stronger current is required in order to reach beyond the kill-zone, which makes the electrodes imprecise – and then other areas in the brain are also affected, which causes side effects. As the new flexible electrodes do not create a kill-zone, it is possible to use a weaker current and thereby obtain more exact stimulation with fewer side effects.
“Our plan is to develop electrodes that are implanted and splay out in the tissue. Each electrode will stimulate a small area. We will be able to see which of the electrodes provide the desired effect and will continue to use them, whereas those that create side effects will be turned off.”
Another interesting area is to study what happens in the brain during chronic pain in order to develop tailored drugs in the future.
“We want to develop a usable model for the testing of pain relief, as today we are not measuring the right things”, says Jens Schouenborg.
In order to get an accurate picture there should be measurements showing how nerve cells in the cerebral cortex process pain signals. The new, flexible electrodes will be a good fit for this purpose.
Text: Eva Bartonek Roxå
The article was first published in Swedish at XXX
Bildtexter:
The electrodes are cut from a thin sheet of gold leaf, which is only four micrometres thick. The flexible gold electrodes are made up of several separate electrodes (the various leads). This technique means the shape of the electrodes can be adapted to a specific need. (To the left in the photo is a coin for size comparison.) Photo: Neuronano Research Centre.
The film shows how an array of electrodes, which are embedded in gelatine, are implanted in the brain. When the array of electrodes is in place, the gelatine dissolves and the electrodes splay out in the tissue. Film: Neuronano Research Centre.